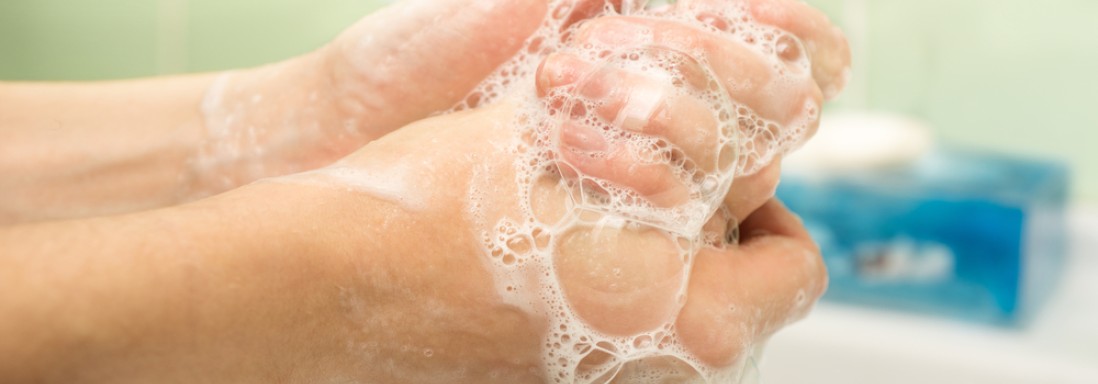
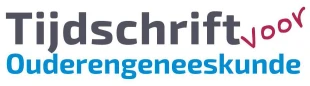
Tijdschrift 2017 | No. 2 - April
Van de redactie
Huidziekten
De huid, het grootste orgaan van ons lichaam. Het heeft een oppervlakte van twee vierkante meter en weegt bij een volwassene al snel zo'n 15 kilo. Het is ook een van de belangrijkste organen, zonder zou je niet kunnen leven. Voor de clinicus practicus is het een voordeel dat eventuele symptomen zich voor iedereen zichtbaar manifesteren. Het is echter aan de hulpverlener om hetgeen dat wordt gezien, professioneel te interpreteren. De interpretatie leidt vervolgens tot een analyse en klinische diagnose. In samenspraak mét de patiënt wordt daarna een besluit genomen over het uit te voeren behandelplan.
Wij hebben als gasthoofdredacteuren voor een geheel eigen opzet van dit themanummer 'Huidziekten' gekozen. Een aantal medische disciplines die allen te maken hebben met huidziekten kregen de gelegenheid een veel voorkomend huidprobleem in de praktijk van de specialist ouderengeneeskunde, vanuit hun geheel eigen professionele invalshoek, te belichten.